Evaluation of Temperature and Pressure for Increased Resolution for the Separation of Alkanes and Aromatics Using SFC
August 18, 2022
Introduction
As various silica columns were evaluated in another application note, it was determined that smaller pore size led to better resolution, while interestingly producing a decrease in retention of the alkanes. During that column comparison higher back pressures also led to increased retention and increased resolution of alkanes. The JASCO and Princeton 30A silica columns proved to provide the best separation of alkanes and aromatics.
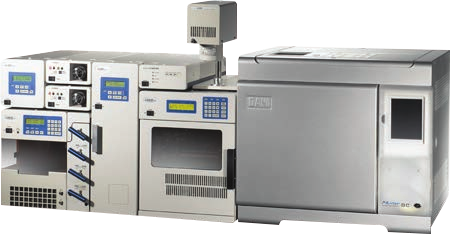
In SFC one additional and unique parameter, back pressure, which is not controlled in HPLC, can be adjusted in SFC to optimize a separation. As with HPLC the column temperature is controlled in SFC, but this change in temperature alters the density of CO2 compared to a change in viscosity in HPLC producing a different effect on the separation in SFC. To further optimize the silica separation for the ASTM D5186 and 6550 fuel analysis methods, pressure and temperature were evaluated below to determine their effect on the resolution.
Experimental
Equipment | Conditions | ||
---|---|---|---|
CO2 Delivery Pump: | PU-2080-CO2 | Column: | JASCO Silica 4.6x250mm, 5um |
Autosampler: | XLC-3159AS | CO2 Flow rate: | 2.0 mL/min |
Column Oven: | CO-2060 | Column temp.: | Various |
FID: | DANI Oven-FID | Back Pressure: | Various |
Back Pressure Regulator: | BP-2080 | FID Splitter: | 200 oC |
FID Detector: | 350 |
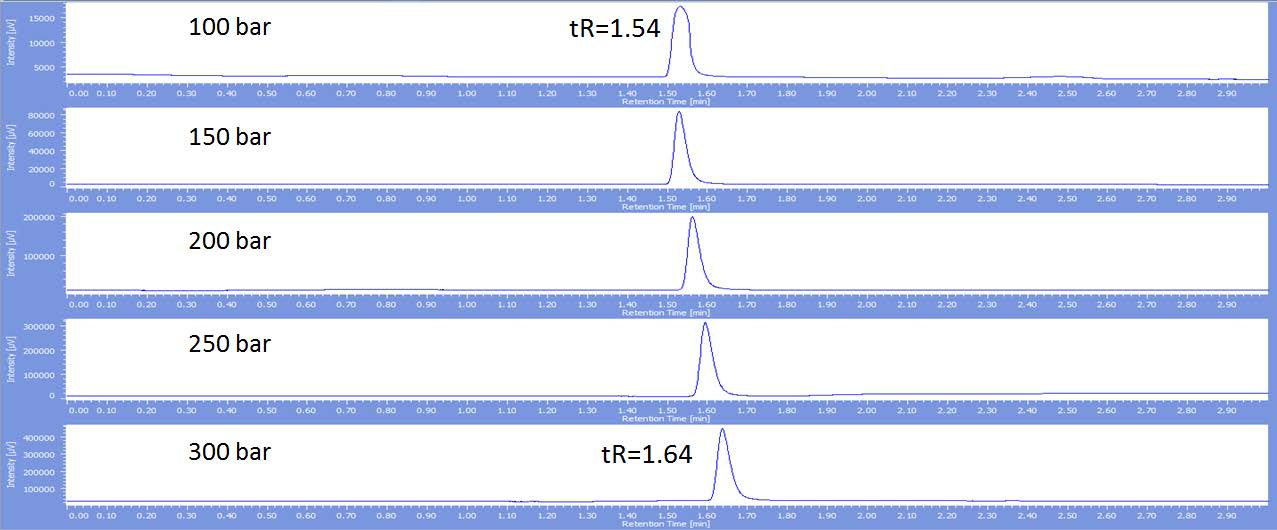
Figure 1 and figure 2 show that the retention time increases as the back pressure is increased for both hexadecane and cyclohexane. However the magnitude of this increase is different for each. Hexadecane at 100bar elutes at 1.54 minutes and has poor peak shape, while cyclohexane elutes earlier at 1.46 minutes. The peak shape sharpens as the back pressure is increased for hexadecane, but notice the retention time only increases to 1.64 minutes at 300 bar compared to later cyclohexane elution at 1.70 minutes. Effectively if these two compounds were in the same mixture their elution order would reverse showing the importance of the back pressure.
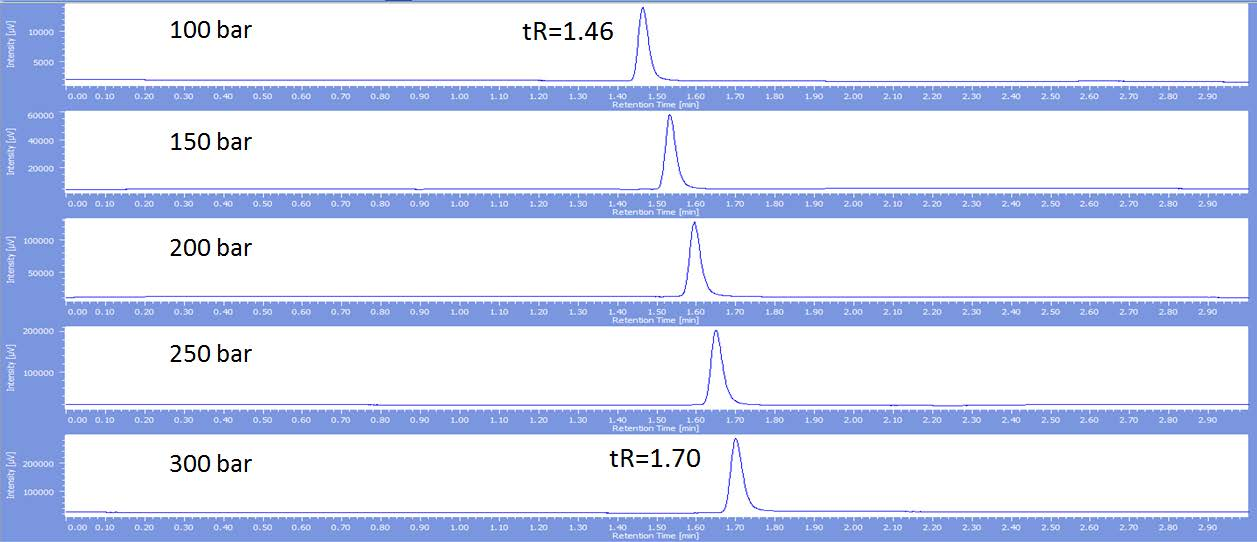
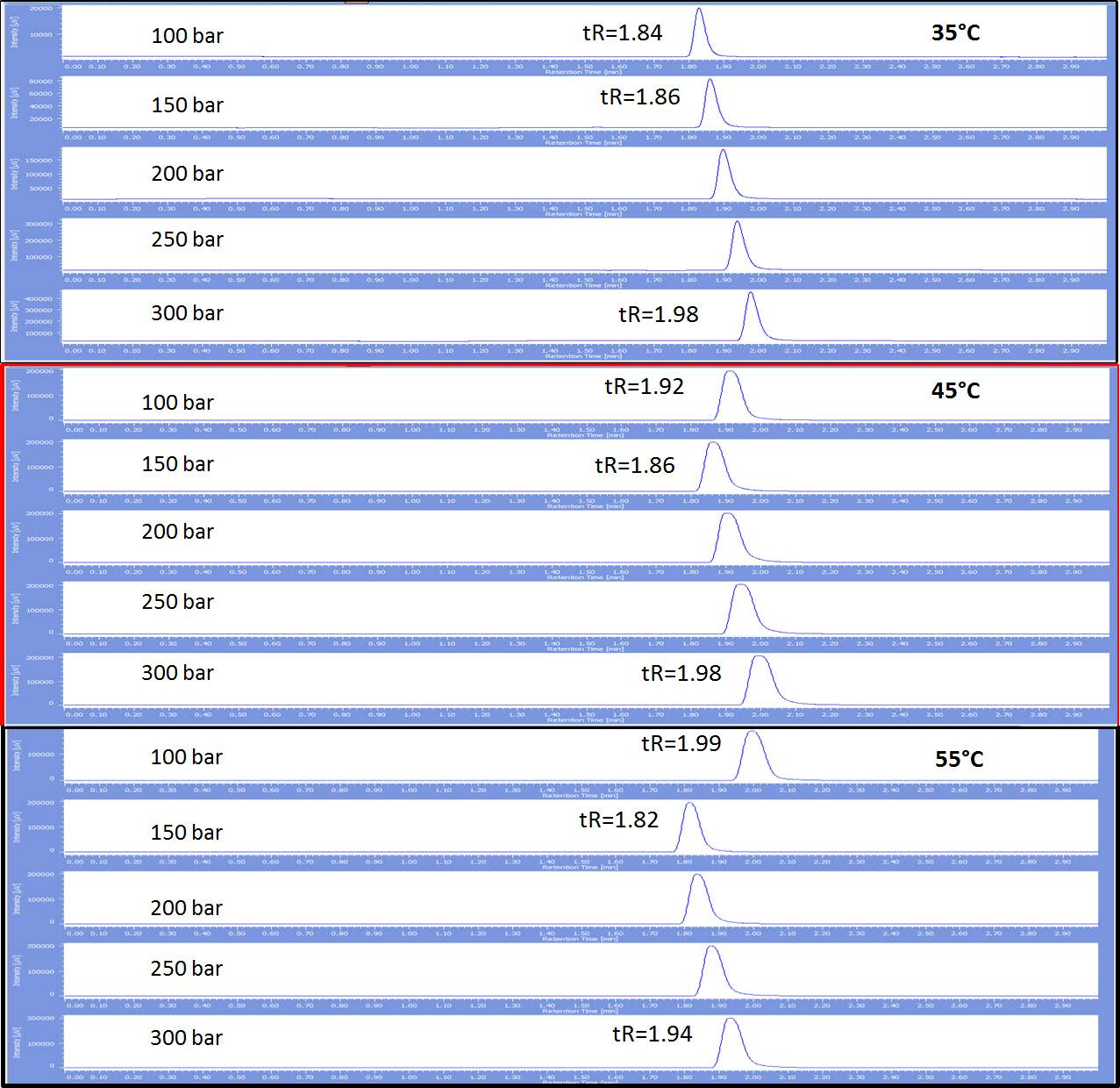
Figure 3 shows the retention behavior of toluene at various temperatures and various temperatures. The retention time does not vary between 35°C and 45°C with the exception at 100 bar yielding a slightly longer retention. Although the retention times do not vary, the peak shape is certainly sharper at 35°C. At 55°C the peak shape mimics that broader peak shape seen at 45°C, but the retention time decreases slightly, with the exception of 100 bar where the retention time increases. This same data set was repeated using benzene as the sample and the exact same retention behavior was displayed. With benzene, the peak broadening was also evident at 45°C and 55°C compared to 35°C, but was much less significant.
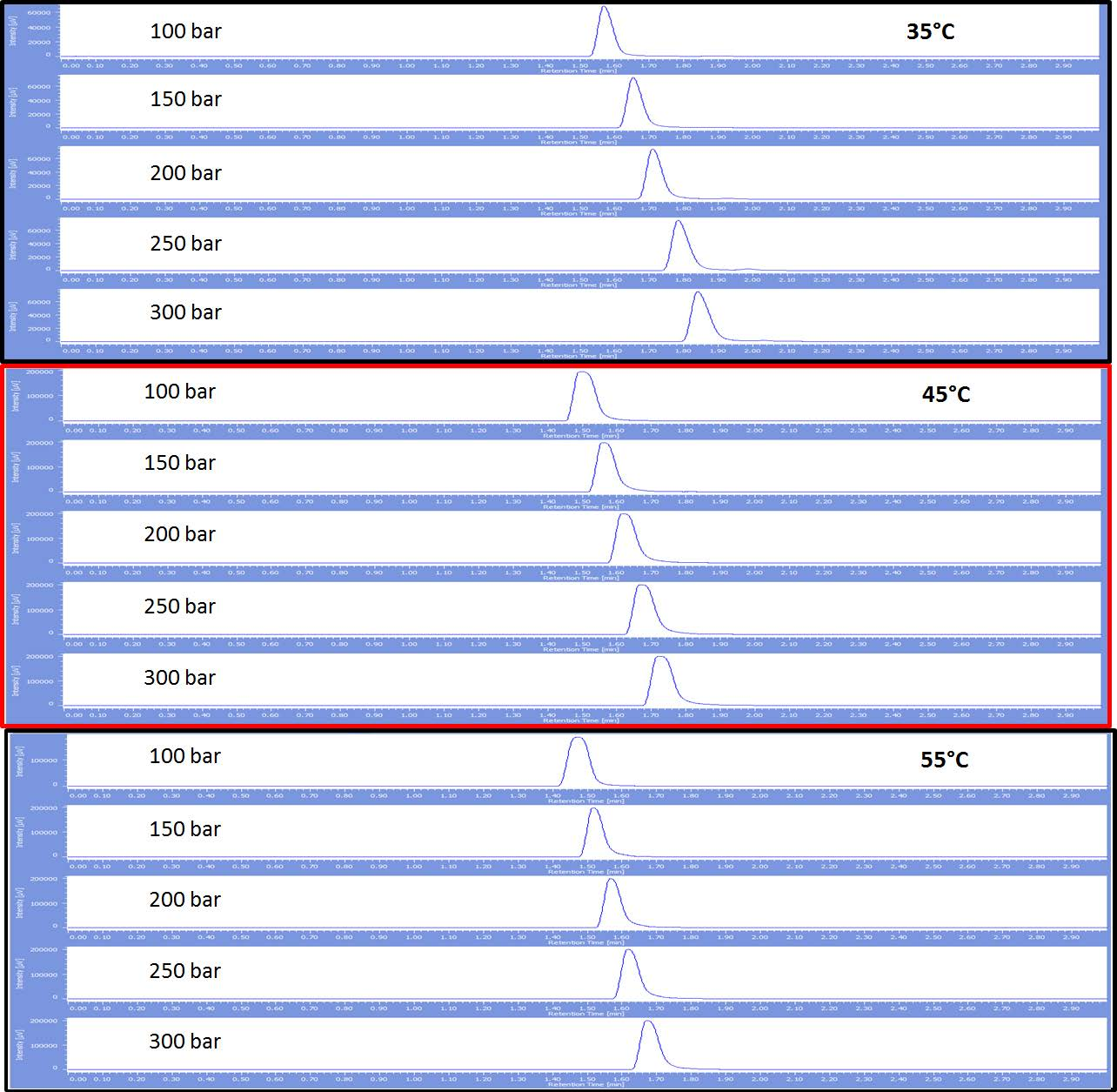
Figure 4 displays the chromatograms of toluene at the same back pressures and temperatures as seen in figure 3, but the mobile phase is 90% CO2 :10% methanol. The trend is identical to that seen in figure 3 with increased retention as the pressure is increased as well as slight peak broadening at 45°C and 55°C.
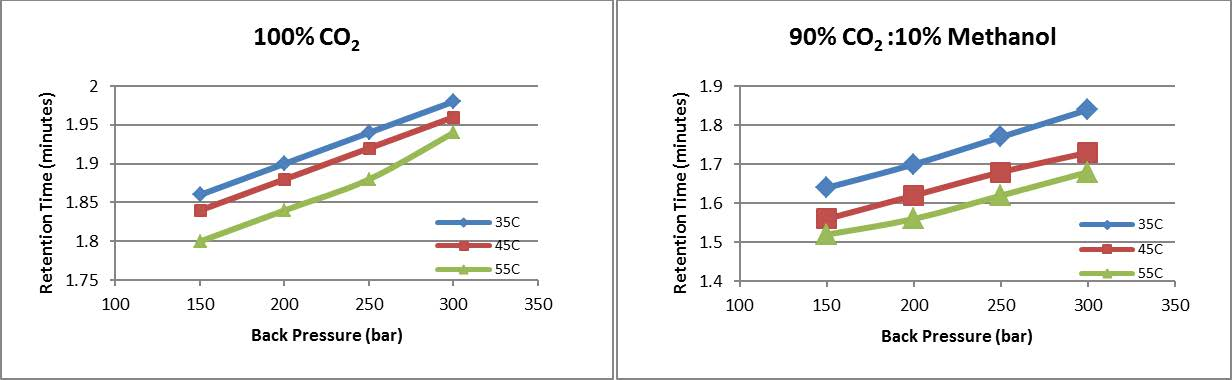
The retention time increases as the pressure is increased from 150 bar up to 300 bar with both 100% CO2 and 90% CO2 : 10% methanol as plotted in figure 5 and seen in figures 3 and 4. The retention time trend is identical for both with 10% methanol as expected producing earlier elution. At 100 bar, however, the retention time does not follow the trend when the mobile phase is 100% CO2 and toluene has longer retention as the temperature is increased from 35°C to 45°C to 55°C. This suggests that there is a significant difference in the mobile phase (100% CO2) density at 100bar for 35°C and 55°C producing very different retention times. The presence of 10% methanol, which is in the liquid phase, inhibits the density change leading to retention times that follow the trends seen at higher pressures.
Conclusion
This application has not only illustrated how the unique parameter of back pressure plays a significant role in retention time, but also how it can be utilized to optimize a separation when resolution is minimal. Both alkanes and aromatics were more retained at higher pressures, but higher temperatures had the opposite effect on retention and also led to peak broadening. These significant effects from back pressure and temperature provide a user the ability to tune a separation depending on to which class of compounds they are looking to maximize the resolution.
Featured Products:

Evaluation of Temperature and Pressure for Increased Resolution for the Separation of Alkanes and Aromatics Using SFC
Introduction
As various silica columns were evaluated in another application note, it was determined that smaller pore size led to better resolution, while interestingly producing a decrease in retention of the alkanes. During that column comparison higher back pressures also led to increased retention and increased resolution of alkanes. The JASCO and Princeton 30A silica columns proved to provide the best separation of alkanes and aromatics.
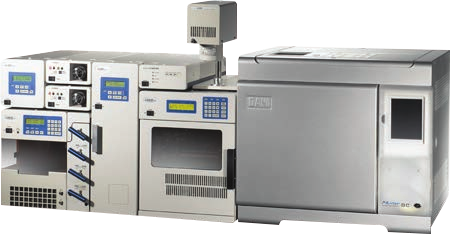
In SFC one additional and unique parameter, back pressure, which is not controlled in HPLC, can be adjusted in SFC to optimize a separation. As with HPLC the column temperature is controlled in SFC, but this change in temperature alters the density of CO2 compared to a change in viscosity in HPLC producing a different effect on the separation in SFC. To further optimize the silica separation for the ASTM D5186 and 6550 fuel analysis methods, pressure and temperature were evaluated below to determine their effect on the resolution.
Experimental
Equipment | Conditions | ||
---|---|---|---|
CO2 Delivery Pump: | PU-2080-CO2 | Column: | JASCO Silica 4.6x250mm, 5um |
Autosampler: | XLC-3159AS | CO2 Flow rate: | 2.0 mL/min |
Column Oven: | CO-2060 | Column temp.: | Various |
FID: | DANI Oven-FID | Back Pressure: | Various |
Back Pressure Regulator: | BP-2080 | FID Splitter: | 200 oC |
FID Detector: | 350 |
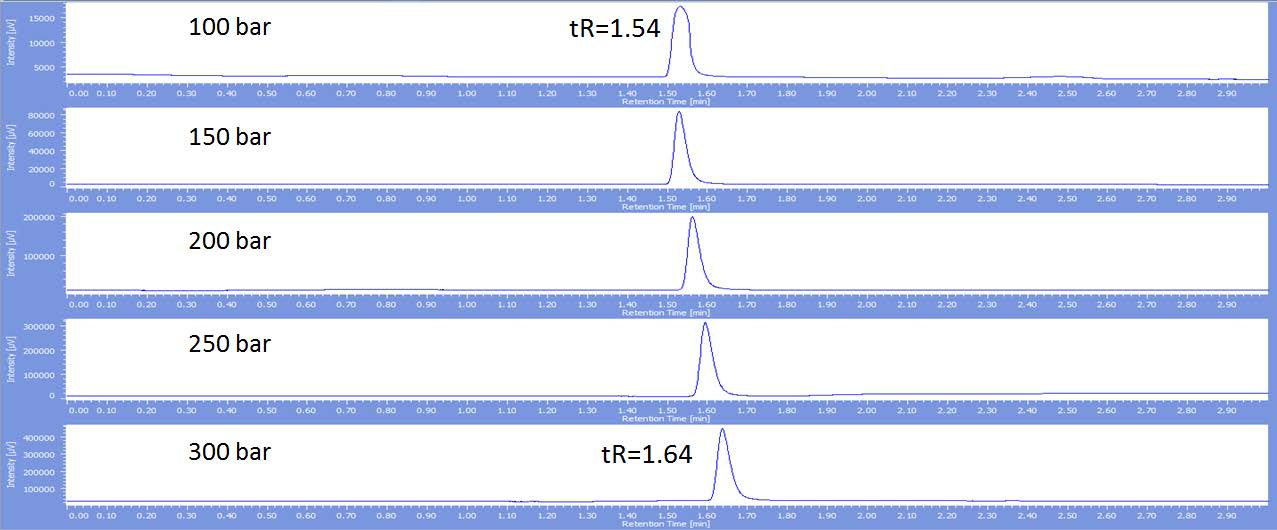
Figure 1 and figure 2 show that the retention time increases as the back pressure is increased for both hexadecane and cyclohexane. However the magnitude of this increase is different for each. Hexadecane at 100bar elutes at 1.54 minutes and has poor peak shape, while cyclohexane elutes earlier at 1.46 minutes. The peak shape sharpens as the back pressure is increased for hexadecane, but notice the retention time only increases to 1.64 minutes at 300 bar compared to later cyclohexane elution at 1.70 minutes. Effectively if these two compounds were in the same mixture their elution order would reverse showing the importance of the back pressure.
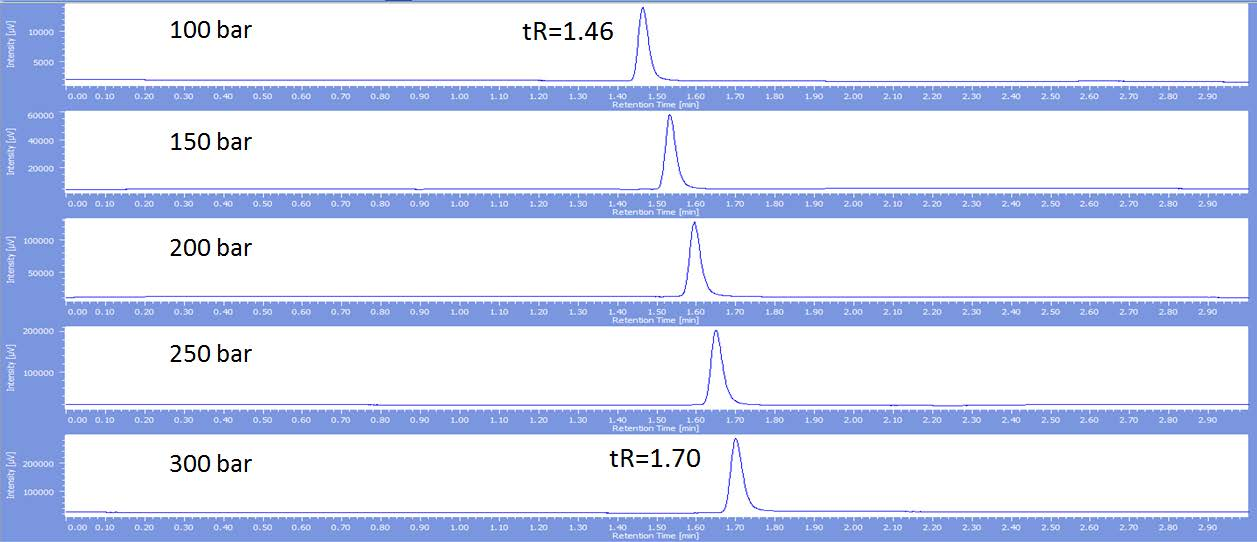
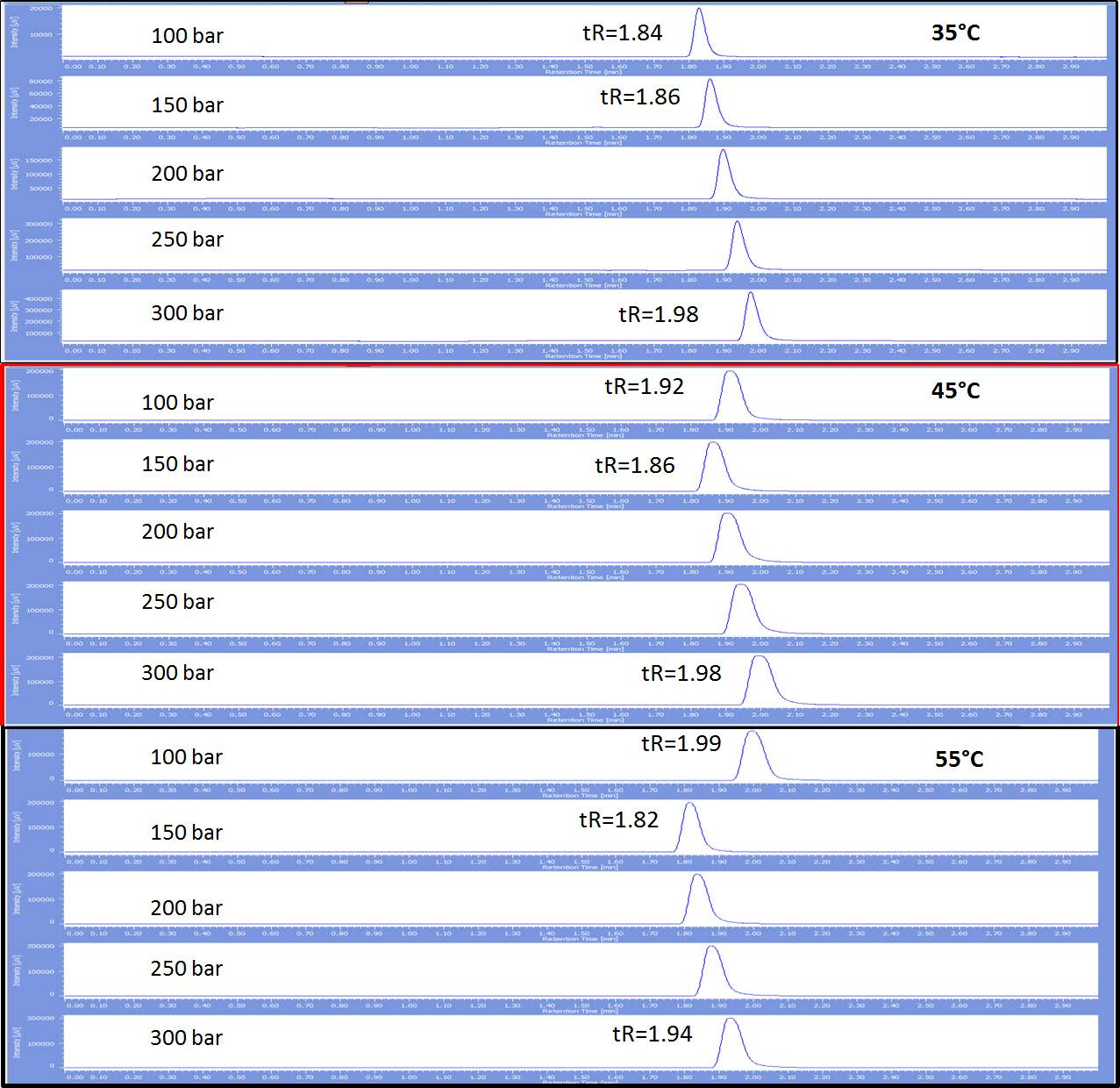
Figure 3 shows the retention behavior of toluene at various temperatures and various temperatures. The retention time does not vary between 35°C and 45°C with the exception at 100 bar yielding a slightly longer retention. Although the retention times do not vary, the peak shape is certainly sharper at 35°C. At 55°C the peak shape mimics that broader peak shape seen at 45°C, but the retention time decreases slightly, with the exception of 100 bar where the retention time increases. This same data set was repeated using benzene as the sample and the exact same retention behavior was displayed. With benzene, the peak broadening was also evident at 45°C and 55°C compared to 35°C, but was much less significant.
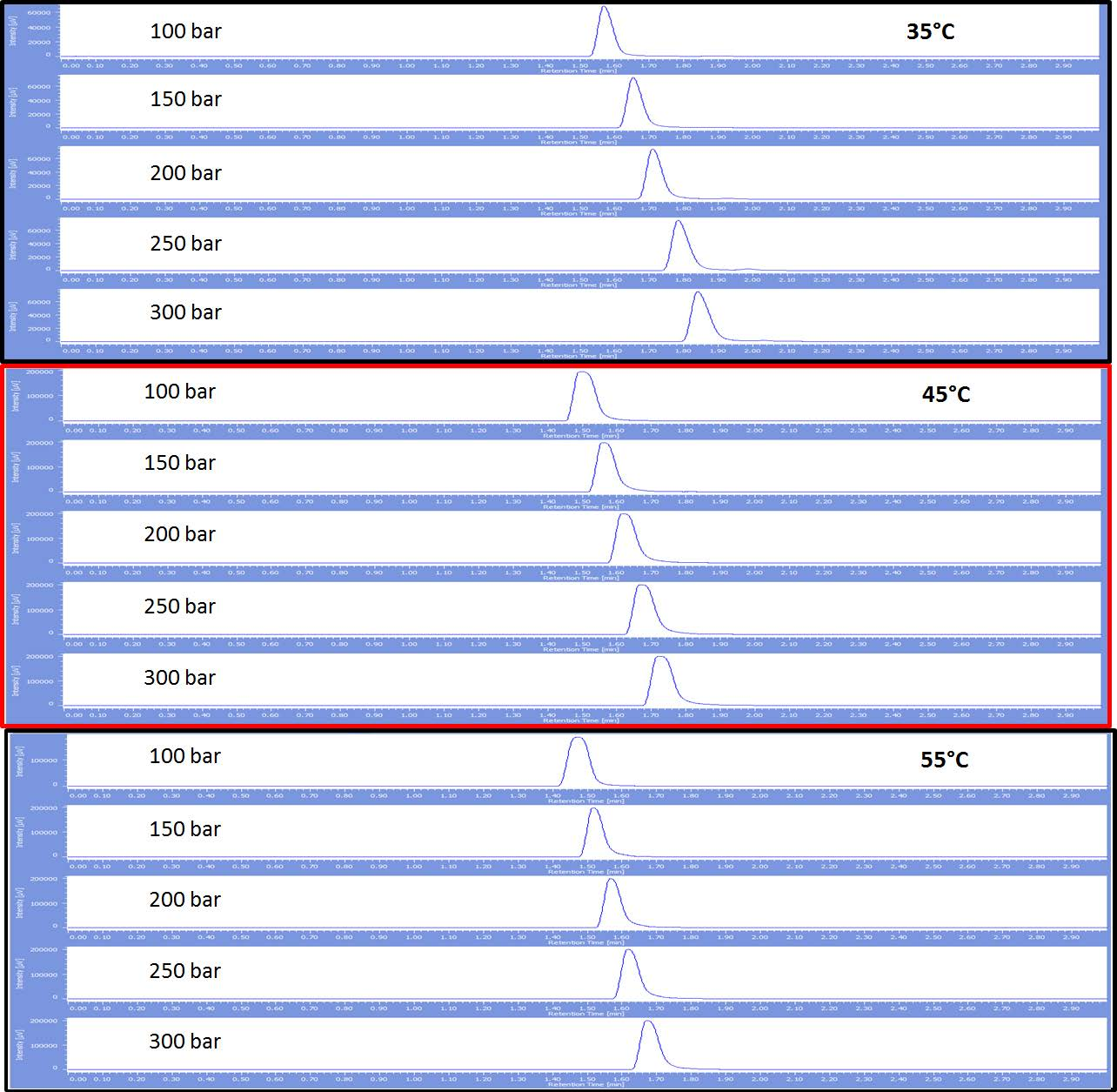
Figure 4 displays the chromatograms of toluene at the same back pressures and temperatures as seen in figure 3, but the mobile phase is 90% CO2 :10% methanol. The trend is identical to that seen in figure 3 with increased retention as the pressure is increased as well as slight peak broadening at 45°C and 55°C.
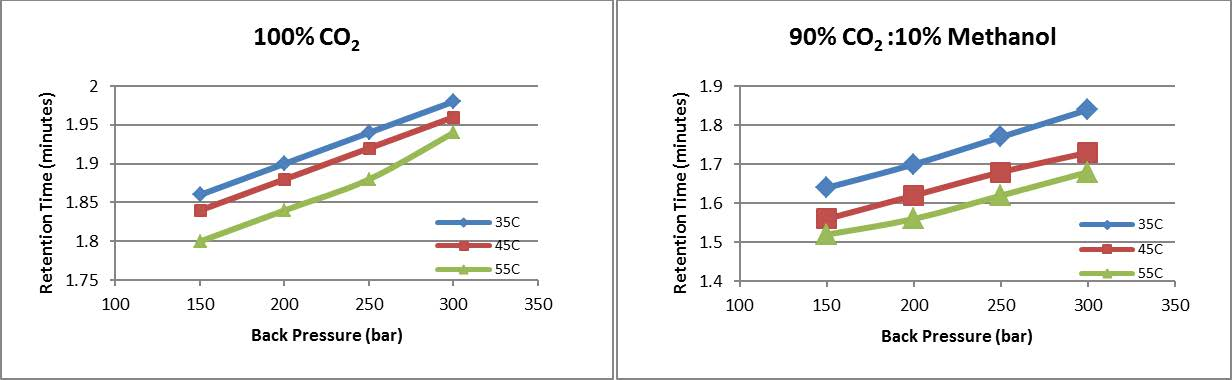
The retention time increases as the pressure is increased from 150 bar up to 300 bar with both 100% CO2 and 90% CO2 : 10% methanol as plotted in figure 5 and seen in figures 3 and 4. The retention time trend is identical for both with 10% methanol as expected producing earlier elution. At 100 bar, however, the retention time does not follow the trend when the mobile phase is 100% CO2 and toluene has longer retention as the temperature is increased from 35°C to 45°C to 55°C. This suggests that there is a significant difference in the mobile phase (100% CO2) density at 100bar for 35°C and 55°C producing very different retention times. The presence of 10% methanol, which is in the liquid phase, inhibits the density change leading to retention times that follow the trends seen at higher pressures.
Conclusion
This application has not only illustrated how the unique parameter of back pressure plays a significant role in retention time, but also how it can be utilized to optimize a separation when resolution is minimal. Both alkanes and aromatics were more retained at higher pressures, but higher temperatures had the opposite effect on retention and also led to peak broadening. These significant effects from back pressure and temperature provide a user the ability to tune a separation depending on to which class of compounds they are looking to maximize the resolution.