History of Supercritical Fluid Chromatography
In 1822, French physicist Charles Cagniard de la Tour put a liquid and a flint ball into a Papin pressure vessel constructed using a rifle and heated the liquid in a sealed cannon. He then conducted an experiment to determine whether a product was produced.1) When the container was shaken, a splashing sound was heard as the ball penetrated the liquid-gas interface. However, when the container was heated to a temperature sufficiently higher than the boiling point of the liquid, the sound disappeared. He postulated that this was because the liquid and gas densities in the container became equal, so that a single-phase was effectively formed. This represented the first discovery of what are now referred to as supercritical states and critical points.
Following this discovery, supercritical fluids became the subject of fundamental physicochemical research to determine the change in the state of materials and their physical properties under supercritical conditions. However, no practical applications immediately emerged. Then, in 1879, Hannay and Hogarth reported that supercritical fluids possessed excellent solvent properties,2) and a second wave of research began.
1) C. Cagniard de la Tour, Ann. Chim. Phys. 21, 127 (1822)
2) J. B. Hannay and J. Hogarth, Proc. R. Soc. London, 29,324 (1879)
Supercritical Fluid Chromatography Explained
Substances can be in a solid, liquid, or gaseous state depending on the temperature and pressure conditions. For example, water is a liquid at room temperature and pressure, but at atmospheric pressure, it changes to vapor (gas) at 100 degrees C and ice (solid) at 0 degree C. When water is placed inside a sealed container and subjected to a vacuum as shown in Fig. 1, some of the water evaporates and the remainder remains in a liquid phase . When the water vapor pressure reaches a certain value, the evaporation rate becomes equal to the condensation rate. This is referred to as the saturated water vapor pressure, and depends on temperature.
As the vessel is heated, the liquid water expands and evaporates, so that its density is reduced. This leads to an increase in the density of the vapor phase. If the temperature exceeds 374 degrees C and the pressure exceeds 22.06 MPa, the density of the liquid and vapor phases become the same, making it impossible to distinguish between liquid water and water vapor. The water in this state will not become liquid even if the pressure is increased further. In other words, it’s like a dense gas that doesn’t liquefy.
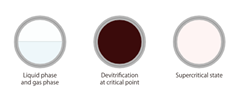
Such a state is called a supercritical state, and a substance in a supercritical state is called a supercritical fluid. The temperature and pressure at which a substance becomes supercritical are called the critical point, the temperature is called the critical temperature, and the pressure is called the critical pressure. Fig. 3 shows a phase diagram for an arbitrary substance, and Table 1 shows the critical temperature and critical pressure for various real compounds.
Critical temperature and critical pressure for various compounds
Compound | Critical temperature (℃) | Critical pressure (MPa) |
NH2 | 132 | 11.28 |
CO2 | 31 | 7.38 |
N2O | 36 | 7.24 |
H2O | 374 | 22.06 |
C3H8 | 97 | 4.25 |
C6H14 | 234 | 2.97 |
CH3OH | 239 | 8.09 |
C2H5OH | 243 | 6.38 |
C6H5CH3 | 318 | 4.11 |
The density of a supercritical fluid is close to that of a liquid, and such a fluid is a highly effective solvent. However, the viscosity of a supercritical fluid is close to that of a gas, and the diffusion coefficient of a substance in a supercritical fluid is about halfway between that in a liquid and in gas. In general, supercritical fluids exhibit properties that are intermediate between those of liquids and gases, and these properties are greatly influenced by temperature and pressure. As a result, supercritical fluids are used in a variety of applications such as mobile phases in chromatography, extraction agents, and chemical reaction solvents.
Comparison of phases
Gas | Critical state | Liquid | |
Density [kg/cm3] | 1 | 100 – 1000 | 1000 |
Viscosity [mPa·s] | 0.01 | 0.1 | 1 |
Diffusion coefficient [m2/s] | 10-5 | 10-5 – 10-8 | 10-10 |
Thermal conductivity x10-3 [W/(m・K)] | 5 – 30 | 20 – 150 | 50 – 200 |
All substances are in a supercritical state above the critical point, but those with high critical pressure and critical temperature are not practical. Carbon dioxide is a commonly used supercritical fluid since its critical temperature is just 31.1 degrees C and its critical pressure is only 7.38 MPa. It offers the following advantages:
- It is chemically inert and nontoxic.
- It is non-flammable.
- It is non-polar and dissolves oils and fats well.
- Since carbon dioxide is released as a gas at normal temperatures and pressures, solvent removal is easy, allowing more accurate component concentrations to be determined.
- High-purity carbon dioxide can be obtained at a low price, so low running costs can be realized.
- Since carbon dioxide emitted from petrochemical factories is collected, refined, and used, it does not increase carbon dioxide emissions.
Supercritical fluid chromatography (SFC) uses a supercritical fluid as the mobile phase.
Supercritical fluids are more than an order of magnitude less viscous than liquids, and the diffusion coefficient of substances in supercritical fluids is several hundred times greater than that in liquids. For this reason, SFC can achieve high separation efficiency even when the flow rate of the mobile phase is increased. Therefore, SFC allows substances to be separated at a higher speed than is possible using high-performance liquid chromatography (HPLC) with a liquid as the mobile phase. In addition, the density and properties of supercritical fluids change with pressure and temperature, allowing the separation rate to be adjusted. Furthermore, by mixing an auxiliary solvent (modifier) such as alcohol with the supercritical fluid, it is possible to change the properties of the entire mobile phase and adjust the retention time and separation.
In general, the substance used as the mobile phase in SFC is carbon dioxide. Carbon dioxide has the same polarity as hexane and is suitable for the separation of non-polar substances. For this reason, a column with silica gel as a packing material shows retention behavior similar to that for normal phase chromatography. It is also possible to use packed columns for reversed-phase like chromatography. In this particular case, a modifier is used to separate highly polar substances. In order to separate more highly polar substances, it is necessary to increase the amount of modifier added.
When alcohol is added to carbon dioxide, the critical point of the mixture becomes higher than that for carbon dioxide. For this reason, separation is often performed in subcritical or liquid states where the mobile phase has not reached the supercritical state, but for convenience, it is still referred to as SFC.
Fig. 4 shows the basic flow diagram for SFC. The liquid carbon dioxide is pumped by the liquefied carbon dioxide pump. The temperature and pressure are controlled by the column oven and the back-pressure regulator to bring the mobile phase into a supercritical state. If a modifier is added, the modifier pump is used and the composition ratio of carbon dioxide and modifier can be determined arbitrarily. After passing through the column, the sample goes to the detector. Since the optical detector is generally placed before the back-pressure regulator, a cell with a high-pressure resistance is required.
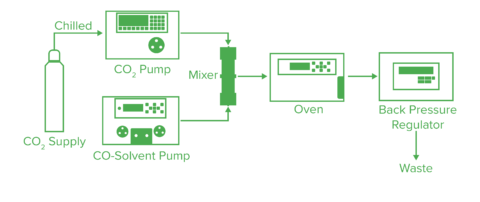
Additional detectors, such as a flame ionization detector, mass spectrometer, and evaporative light scattering detector, can be used with this system. When placing these detectors upstream of the automatic pressure control valve, a splitter is used to introduce a portion of the mobile phase into the detector. The solvent can then be added using the ionization accelerator feed pump for the purpose of reliably introducing the mobile phase to the detector and improving the sensitivity. If the flow rate is low, a detector can be placed downstream of the automatic pressure control valve and the entire volume of solution can be introduced.
In semi-preparative / preparative SFC, carbon dioxide is vaporized when the sample is brought to normal pressure, so post-treatment of the solvent after separation is easy.
In some cases, SFC is referred to as unified fluid chromatography or unified chromatography because the mobile phase can be made supercritical, subcritical, or liquid by controlling the pressure, temperature, and modifier ratio.
Traditionally, SFC was considered to be applicable only to chiral and low-polarity components. However, due to recent advances in performance and column types, it is becoming possible to analyze an ever-expanding range of achiral and highly polar compounds.
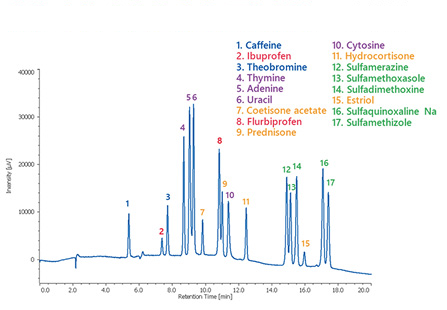
In addition, SFC may allow separation of substances that cannot be separated by gas chromatography (GC) or HPLC. Furthermore, with the advent of preparative SFC and SFC-MS, SFC is attracting attention as a third chromatography method following GC and HPLC.
Supercritical Fluid Extraction SFE
Supercritical fluid extraction (SFE) is a method in which supercritical fluid as an extraction medium is added to substances containing target components, and extraction is performed based on differences in solubility. In particular, the use of supercritical carbon dioxide as an extraction medium offers many advantages in a variety of fields, including short extraction times, simplified operation, and improved extraction efficiency compared with the organic solvent extraction method. Also, solvent removal following extraction is much easier, allowing more accurate component concentrations to be determined.
Because the critical temperature of carbon dioxide is only 31 °C, extraction is possible close to room temperature. Furthermore, extraction can be carried out in a carbon dioxide atmosphere without oxygen. This means that this method can be used for substances that are temperature-sensitive or easily oxidized. Supercritical carbon dioxide is effective at dissolving lipid-soluble or low-polar compounds, but it can be adapted to the extraction of highly polar components by adding alcohols such as methanol or organic solvents such as acetonitrile and tetrahydrofuran as auxiliary solvents (referred to as entrainers).
In recent years, it has attracted attention as an environmentally friendly extraction method that does not use harmful organic solvents, in line with the concept of Green Chemistry.
Comparison of conventional organic solvent and supercritical carbon dioxide extraction and pretreatment methods
Organic solvent | Disadvantages |
Soxhlet extraction & purification | Uses harmful organic solvents |
Solvent extraction & purification | Process is complicated and time-consuming Long pre-processing time Higher sample concentration required Trace residual solvent |
Supercritical carbon dioxide | Advantages |
Carbon dioxide | Uses safe carbon dioxide |
Carbon dioxide & ethanol, etc. | Simple process that can be automated Fast pre-processing and extraction Selective extraction possible Online SFE-SFC Prevention of sample oxidation, operation at low temperature |
Applications of supercritical carbon dioxide extraction
Field | Material | Purpose |
Food | Coffee beans | Decaffeinated |
Tea | Flavor ingredient | |
Hop | Hop extract | |
Red pepper | Spicy extract, pigment | |
Fish meal | Fish oil | |
Fish oil | EPA, DHA | |
Plant | Essential oils, pigments, medicinal ingredients | |
Vegetable oil | Vitamin E | |
Pharmaceutical | Natural products | Medicinal ingredients, bioactive substances |
Tablet | Residual solvent | |
Chemical | Coal, oil, energy Plastic, rubber, polymer Metal Inorganic compounds and ceramics Aerogel Electrical / electronic parts |
Low boiling point component |
Polymerization solvent | ||
Monomer | ||
Oligomer | ||
Impurities | ||
Nasty smell | ||
Developing agent | ||
Degreasing | ||
Dehydration | ||
Solvent removal | ||
Washing | ||
Others | Cigarettes | Nicotine |
Soil | Organic matter |
Supercritical carbon dioxide extraction system
The basic configuration and flow diagram of the SFE system is shown in Fig. 6. The system is configured with a liquefied carbon dioxide feed pump, an entrainer feed pump, a high-pressure vessel in the oven, and a back-pressure regulator valve for controlling the extraction pressure.
Fig. 6 Configuration of supercritical fluid extraction system
For the high-pressure vessel, a column-type or cup-type design can be selected according to the state of the extracted sample, and various capacities are available. The entrainer solvent type and percentage, pressure, temperature, and time are all parameters that are controlled during the extraction. Each of these can be changed to optimize the extraction efficiency. It is also possible to attach a viewing cell that allows real-time observation of sample extraction and reaction processes under supercritical conditions through the observation window.
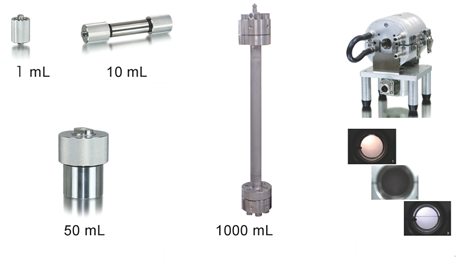