IR spectrometer contains a Michelson interferometer splits a collimated beam of polychromatic infrared light into two different optical paths that cause constructive and destructive interference based on the position of a stationary and moving mirror. This interference waveform (interferogram) is converted from the time domain to the frequency domain via a Fast Fourier Transform (FFT). The final spectrum is obtained by separating a broad spectrum of IR radiation into individual frequencies using the precisely known wavelength of a timing laser.
• How does an interferometer work?
• How is a digital interferogram acquired?
• Instrumentation of a IR Spectrometer
How does an interferometer work?
Monochromatic light is a superposition of waves of the same frequency. The light intensity is a maximum when the individual waves have the same phase and constructively interfere with each other (Figure 1a). The light intensity decreases as the phase difference becomes larger and reaches a minimum when the individual waves are out of phase and destructively interfere with each other (Figure 1b). In a FTIR spectrometer, this phase difference is varied using a dual light path and two mirrors: one mirror that is fixed in place and another mirror that moves at a constant speed.
For a monochromatic light source, a plot of the detected light intensity against time (or position of the moving mirror) appears as a sinusoidal wave (Figure 2a). The time domain signal of this waveform is converted to the frequency domain via a Fast Fourier Transform (FFT), producing a single peak at the frequency of the monochromatic light. For a bichromatic light source, containing two waves of different frequencies, a more complicated waveform is produced. Taking a Fourier transform of this waveform produces two peaks corresponding to the two different frequencies of light (Figure 2b). For a continuous light source, its spectrum corresponds to a superposition of waves of different frequencies, all in phase with each other. Before the mirror is moved (when the time t = 0), there is no phase difference between the optical paths, and the light intensity at all wavenumbers is highest. As the mirror moves (when the time t > 0), destructive interference occurs, and the signal strength gradually converges to zero. The resulting plot of signal intensity against time is referred to as an interferogram. By taking a Fourier transform of the interferogram, the original frequency spectrum can be calculated (Figure 2c).
Frequency Spectrum Obtained from Interferogram
How is a digital interferogram acquired?
FTIR detectors observe interferograms as analog signals, but in order to perform a Fourier transform, the analog signals first need to be converted to digital signals. In a FTIR spectrometer, monochromatic light from a timing laser is used as an internal reference to track the position of the moving mirror using the precisely known wavelength of the laser. The intensity of the output interference signal is measured as a function of time. Each data point (or digital signal) in the infrared interferogram is taken when the timing laser interferogram has neither constructive nor destructive interference, allowing for an interferogram to be obtained at equal intervals (Figure 3). Helium-Neon (HeNe) lasers are the industry standard due to their excellent wavelength stability of 632.8 nm compared to solid-state or diode lasers. This laser stability allows for spectral additions, library searches, and other functions that require high wavenumber accuracy (Conne’s Advantage).
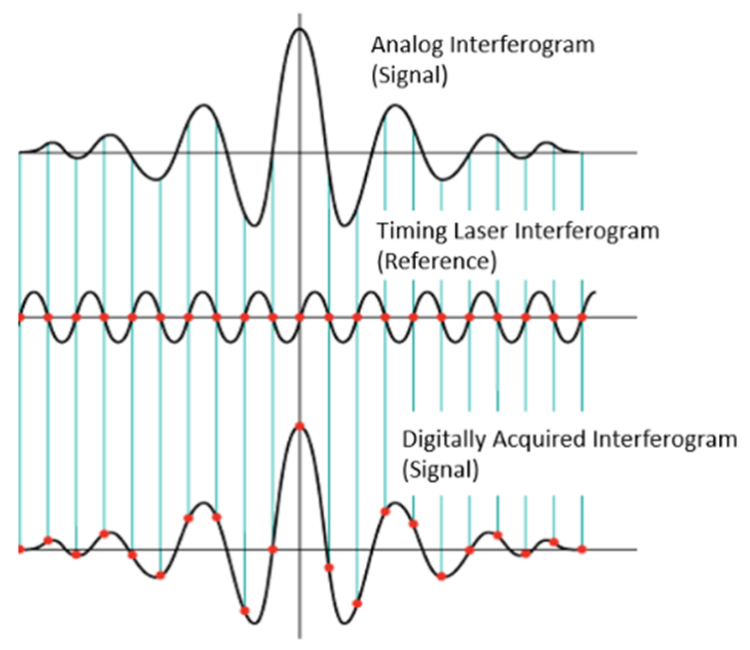
Instrumentation of a IR Spectrometer
A IR spectrometer consists of four major parts: a light source, an interferometer, a sample chamber, and a detector (Figure 4).
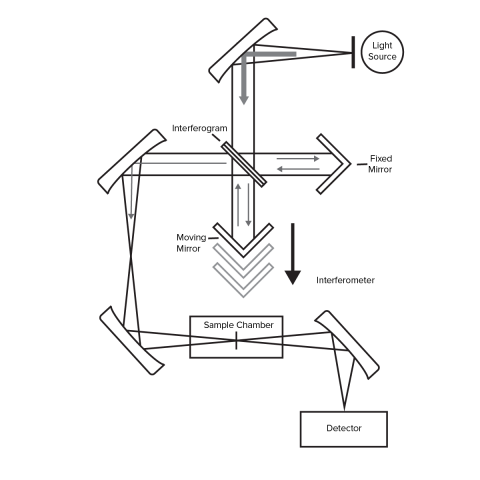
IR Spectrometer Light Sources
The light source for a IR spectrometer is typically a broadband emitter, such as a mid-IR ceramic source (50 – 7,800 cm-1), a near-IR halogen lamp (2,200 – 25,000 cm-1), or a far-IR mercury lamp (10 – 700 cm-1). JASCO FTIR spectrometers come standard with a high-intensity ceramic source.
IR Spectrometer Michelson Interferometer
The interferometer is the heart of a IR spectrometer and consists of a beam splitter, a stationary mirror, and a moving mirror. The beam splitter is a semi-transparent mirror that splits a collimated beam of light into two different optical paths. Half of the light is transmitted to the moving mirror while the other half of the light is reflected to the stationary mirror. The two light beams are reflected from the moving and stationary mirror where they are recombined back at the beam splitter before passing through the sample chamber and onto the detector. The difference in the path of the mirrors causes constructive and destructive interference over the course of time it takes for the moving mirror to make a pass. The power of the beam (Pin) is attenuated by the sample due to absorbance by the sample (Pout), Figure 4. The relationship between power, transmittance, and absorbance can be seen in Figure 5.
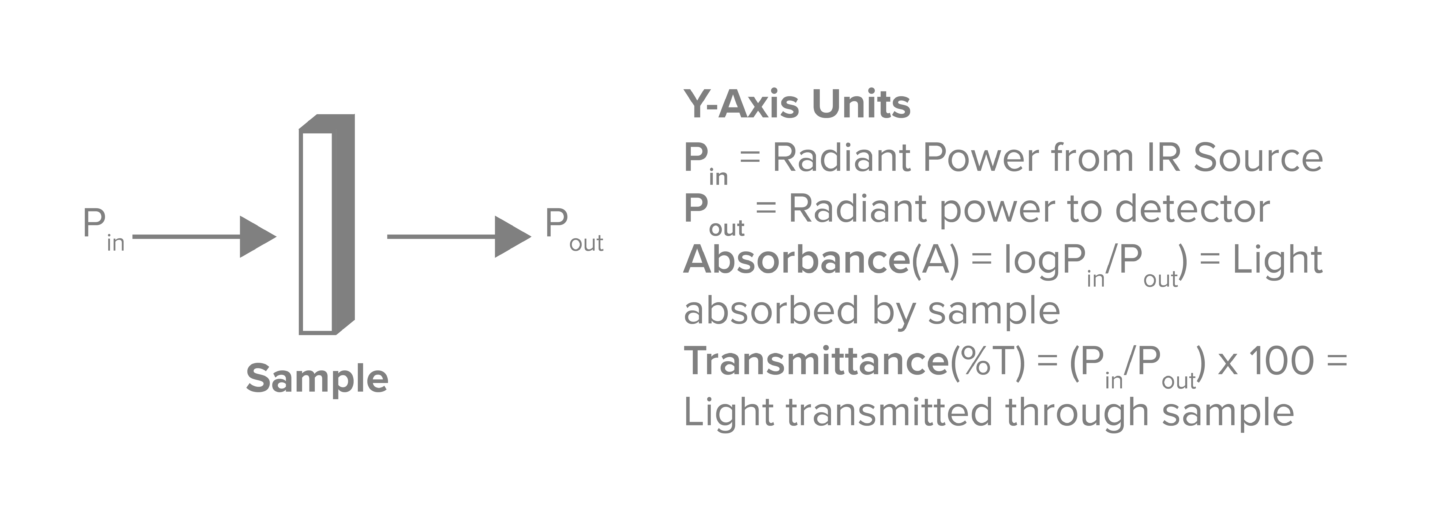
IR Spectrometer Beamsplitters
In many IR spectrometers, the beam splitter is placed at 45° to the incident beam, but for high throughput applications, a low angle interferometer (such as the 28° design used by JASCO) is preferred as the S and P polarizations converge close to Brewster’s Angle. Common beam splitter materials are KBr (375 – 12,000 cm-1) for mid-IR, Quartz (4,000 – 25,000 cm-1) for near-IR, and Mylar (30 – 680 cm-1) for far-IR. JASCO FTIR spectrometers come standard with a Germanium coated KBr beam splitter.
IR Spectrometer Detectors
FTIR detectors are used to measure the transmitted or reflected light from a sample and convert it into an electrical signal. The type and material of the detector will determine the sensitivity and wavelength range of the data that can be acquired. Common FTIR spectrometer detectors include room temperature DLaTGS (220 – 15,000 cm-1) for routine analysis, liquid nitrogen cooled MCT (450 – 12,000 cm-1) for high sensitivity applications, silicon photodiodes (10,000 – 25,000 cm-1) for visible and near-IR measurements, and silicon bolometers (10 – 650 cm-1) for far-IR measurements. JASCO FTIR spectrometers come standard with a DLaTGS detector and have the option to install a second detector, such as a liquid nitrogen cooled MCT detector.
A DLaTGS (Deuterated L-Alanine doped Triglycine Sulphate) detector is a pyroelectric detector that generates an electrical signal based on temperature changes that are proportional to the intensity of IR radiation hitting the detector (Figure 6). DLaTGS detectors are standard for routine FTIR measurements since they have a linear response over a wide wavenumber range, are inexpensive, and can be used at ambient temperature.
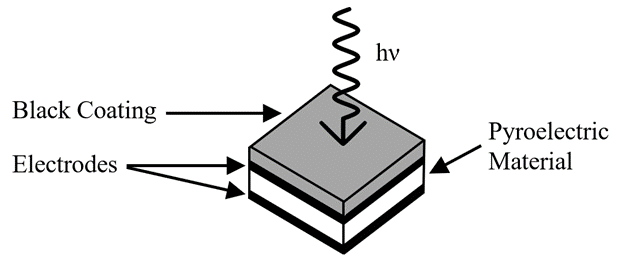
A MCT (Mercury Cadmium Telluride) detector is a semiconductor detector that generates an electrical signal based on the energy of excited electrons in the conduction band proportional to the intensity of IR radiation hitting the detector (Figure 7). MCT detectors are preferred over DLaTGS detectors for samples that require high sensitivity or speed since they produce a spectrum with a higher S/N ratio; however, MCT detectors have a nonlinear response over a reduced wavenumber range, can be easily saturated due to their sensitivity, are more expensive, and require liquid nitrogen cooling to reduce thermal noise.
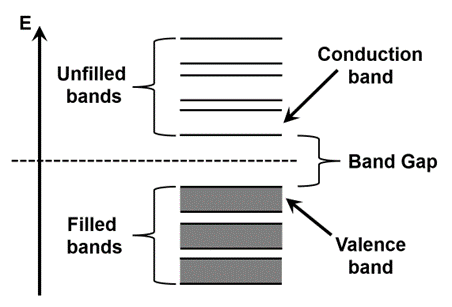
Comparison of IR Spectrometer Optical Components
Further Reading on FTIR Spectroscopy
-
Introduction to FTIR Spectroscopy
FTIR Spectroscopy, Fourier-transform infrared spectroscopy, is concerned with the vibration of molecules.
-
Sampling Techniques for FTIR Spectroscopy
Fourier transform infrared (FTIR) spectroscopy is a widely used analytical technique to identify and quantify samples based on their vibrational spectra.